Published date:
Statement on Electron-Beam Crosslinking
At Heat Trace, we pride ourselves in manufacturing the most technically adavanced range of electric heat tracing equipment in the world. Established in 1974. Heat Trace Limited is now one of the world’s leading suppliers of electric heat tracing equipment for both process temperature maintenance and freeze protection applications. We have produced a stream of novel patented products – both heating cables and control monitoring equipment, utilising state of the art development and manufacturing systems. As part of our commitment to the quality of the cables we manufacture, we take pride in our use of physical crosslinking of the heating matrix that makes the core of our self-regulating cables.
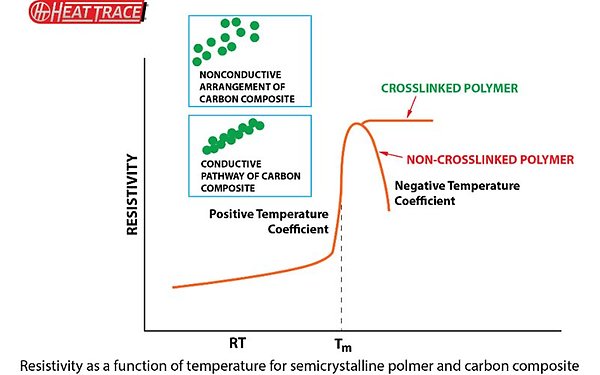
A self regulating heating cable consists of 2 current carrying conductors, separated by a semi-conductive polymer core. It is this semi-conductive core that is the heating element. The polymeric material in the core expands or contracts microscopically in response to temperature variation. The number of electrical paths changes due to the thermal expansion or contraction of the core. The resistance of the core, and hence the power produced by the core, changes as a function of the temperature of the system. The heating core of a self-regulating heating cable is semiconductive in nature. It is made of a composite material with the materials combining in such a way as to generate this semiconductivity. Typically this consists of an electrically-insulating polymer (such as polyethylene), a conductive filler (such as carbon black), and other additives as necessary.¹
In order for this semiconductive core to self-regulate appropriately, and to be safely operated in hazardous areas, the electrical resistance must be tightly controlled.¹,² This is set in the manufacturing process by a combination of formulation – ensuring the correct percentage of conductive material is distributed uniformally through the cable, and by adequate control of production processes, such as extrusion and thermal control.²
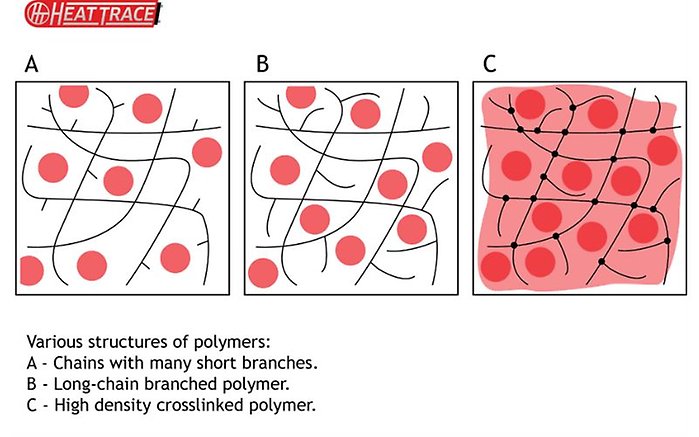
Even with this correctly controlled, however, many of the polymers used for this have a property where they do not hold the carbon particles in fixed locations.³ The carbon is free to slowly migrate through the structure and self-organise. This would happen especially on the repeated heat/cool cycles the heating cables are typically subjected to.³, ⁴
In order to stabilise the structure and, essentially, fix the carbon in place (fixing the electrical resistance at a given temperature), several methods may be used: chemical crosslinking by for example organic peroxides or silanes,³,⁶ generating new covalent bonds; ionic crosslinking, using for example metal ions and carboxylate groups;⁴, ⁵ and physical crosslinking, using gamma rays or electrons.², ⁶
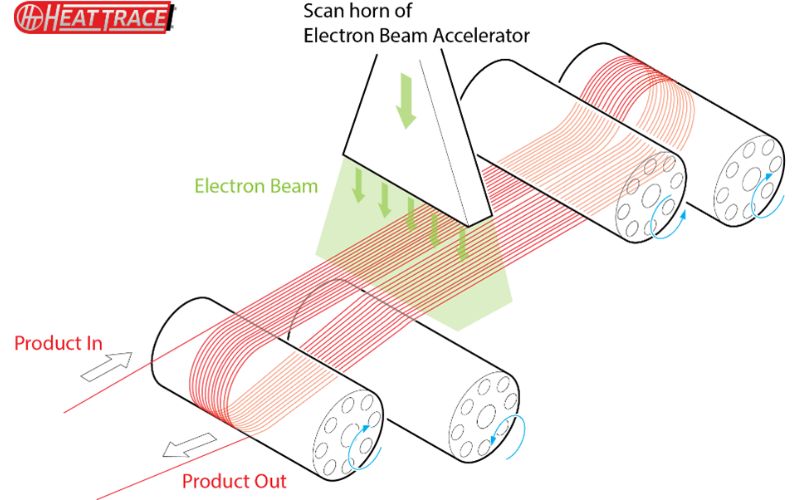
Here at Heat Trace, we believe that physical crosslinking is the safest and most effective method for these products. Chemical crosslinking leaves unwanted chemical residues, catalysts etc. within the product, which may under certain circumstances promote the reverse reaction and un-crosslink the polymer.³, ⁶ This could lead to a lack of product stability and longevity. Ionic crosslinking is only available for certain types of polar polymers such as acrylates and acetates, and is not effective for olefinic or fluorimated polymers often used in self-regulating cables. Ionic crosslinking is also susceptible to reversion to a thermoplastic state under higher temperature exposure.², ⁵, ⁶ Electron-beam crosslinking, however, is a clean process, leaving no residue in the product, has no possibility of reverting to an un-crosslinked state even at elevated temperatures, and works with a wide range of polymers including polyolefins.⁵
Furthermore, as Heat Trace owns and operates our own electron-beam facility at our Helsby UK headquarters, we are fully in control of this process, and hence can optimise and have full oversight, internally verifying product quality, ensuring safe operation and excellent longevity.
References:
- Carbon Black as a Polymer Filler, M. E. Spahr, and R. Rothon, Polymer and Polymeric Composites: A Reference Series, 1-31
- Effect of Colloidal Fillers on the Cross-Linking of a UV-Curable Polymer: Gel Point Rheology and the Winter-Chambon Criterion, Bor-Sen Chiou, Srinivasa R. Raghavan, and Saad A. Khan, Macromolecules 2001, 34, 4526-4533;
- Peroxide Crosslinked Carbon Black Polyethylene Compositions, Eli M. Dannenberg, Merrill E. Jordan and Harvey M. Cole, J. Polymer Science 1985, 31(122), 127-153;
- Detailed understanding of the carbon black–polymer interface in filled rubber composites, Lucas A. Whilke, Christopher G. Robertson, Daniel A. Karsten, Ned J. Hardman, Carbon 2023, 201, 520-528;
- Effect of Carbon Black on Crosslinking, A. N. Gent, J. A. Hartwell, and G. Lee, Rubber Chemistry and Technology, 76(2), 517-532.
- An investigation of chemical crosslinking effect on properties of high-density polyethylene, H. A Khoakdar, J. Morshedian, U. Wagenknecht, S. H. Jafari, Polymer, 44(15), 4301-4309.